KINETIC GLOBAL MODELING FRAMEWORK
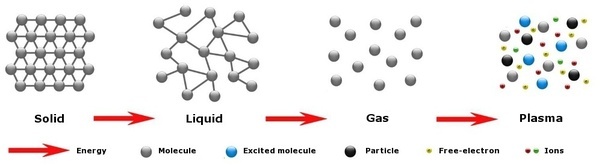
plasma
In the last few decades, advances in computer architecture and scaling have drastically pushed the boundaries of what can be simulated of known physics. The study of plasma in particular requires the combination of electromagnetics, fluid dynamics, atomic and molecular collisions, and statistical mechanics in order to begin describing a physical model. There are three main
components to a computational description of a plasma system: the environment and external forces, dynamics of the particles in response to their surroundings, and composition of the particle ensemble. Due to the high number of particles found even in a low pressure system, plasma simulations are performed using some type of approximating method to reduce the computational complexity as direct solve molecular dynamics simulations are usually not feasible. Fluid and Particle-in-Cell (PIC) methods remain the most common, for macroscopic plasmas, by simplifying the dynamics of individual particles into ‘groups’ while maintaining the complexity of transport and spatial variation. Taking the extreme of low spatial resolution, global (volume-averaged) models reduce all spatial dependence to analytic approximations and provide a focused platform for studying reaction kinetics. Though the lack of spatial variations can lead to inaccurate results with regard to parameters of a specific simulated system, the intention is to study physical trends across variations of inputs. With the greatly reduced computational intensity of these simulations, global models can be used to map out a system’s parameter space either in an exploratory manner or retroactively matching experimental results by iterating through possible input changes. An example of the latter is a “collisional radiative model” , wherein parameters of the global model are adjusted until a simulated spectrum matches that of an experiment. In the context of interest in high fidelity simulations, such as PIC, global models can be used to reduce the included reaction set to aminimized configuration maintaining relevant behavior. The reaction kinetics of a given system describe the overall changes in particle densities and temperatures as a result of various forms of energy transfer. In the context of classical chemistry, this typically involves decomposition, displacement, and synthesis reactions involving molecular and atomic species. Plasma chemistry then expands the number of tracked species to include excited species created through processes such as electron impact ionization and excitation. Reactions involving electrons are herein referred to as ‘plasma phase’ whereas reactions between only heavy species are referred to as ‘gas phase.’ Further effects, such as diffusion or charge species absorption by sheaths, are treated through the use of approximate models. A reaction mechanism is then created by compiling a collection of all relevant reactions and effects involving species of interest, represented by a global model in the form of a coupled set of ordinary differential equations (ODE). While the simplest of models can include a handful of species and reactions, certain systems require hundreds of species and thousands of reactions for an accurate description.