Cone Beam Computed Tomography (CBCT) Uses and Limitations
The discussion on CBCT uses can not be complete without a thorough understanding of the technology’s limitations. Many interpretation errors can occur when the clinician has a wide knowledge of the usefulness of an imaging modality, but fails to comprehend the limitations of the acquisition and reconstruction phases. As discussed previously, the CBCT can provide anatomical accuracy and supplemental information compared to conventional periapical imaging. Velvart et al found the software reconstruction to be useful in locating the inferior alveolar canal and the relationship it holds with posterior teeth prior to surgical procedures. Cottie et al reinforced this while also focusing on the ability to detect cortical plate perforations, root locations and emergence profiles, at reduced radiation exposure compared to conventional computed tomography (CT) imaging. In contrast to a conventional CT image, the CBCT acquisition phase requires only one scan to capture the projection data and then relies on the software to reconstruct the image. The CBCT field of view can also be very limited, down to 4 x 4 cm depending on the manufacturer. The voxel size can also be adjusted and in turn increase the resolution in the required field. Finally, the CBCT uses a pulsating technology during image acquisition to continue to reduce the amount of radiation the patient is exposed to.
In comparison, a typical panoramic radiograph ranges from 4.7-14.9 micro-Sieverts (μSv), a conventional full mouth series ranges from 33-84 μSv, and the CBCT image from 4.7-14.9 μSv. For typical endodontic purposes with a limited field of view, the CBCT acquisition radiation exposure is similar to a panoramic radiograph. When compared to a conventional medical grade CT scan, the CBCT ranges from 2-23% of the radiation exposure. Although any radiation exposure may be considered a relative limitation for the CBCT, it holds a similar dosage and subsequent risk to the patient as other dental imaging techniques. When this information is combined with the dimensional accuracy and reproducibility CBCT offers compared to conventional imaging techniques, the limitation of exposure becomes even more minimal. Cotton et al reinforced minimal radiation limitation and also presented multiple case reports to highlight CBCT usefulness. During retreatment procedures, the CBCT was able to reveal canals missed during prior treatment that was not visible on conventional periapical images. The scan was able to differentiate between endodontic and non-endodontic pathology and led to proper subsequent referral. Resorption can prove to be very challenging to visualize in periapical imaging, but the CBCT was capable of presenting a three-dimensional reconstruction of both internal and external invasive cervical resorption. All of these uses hinge on the elimination of superimposition of anatomical structures.
When this is combined with the ability to navigate through multiple planes, the clinician is able to better understand the anatomical relationships and any existing pathology. Yoshioka et al examined the ability of the CBCT to detect periapical pathology that was difficult to impossible to visualize on periapical images. It has been well established that periapical lesions of cancellous bone only, without cortical plate perforation, are difficult to be visualized on periapical radiography. Teeth that were diagnosed with persistent apical periodontitis clinically, but showed minimal to no signs of a periapical radiographic finding using conventional methods underwent CBCT scans. They found that CBCT could accurately distinguish periapical findings which could not be easily visualized with periapical radiography. Of the 532 teeth, 22% showed radiographic findings that were limited to the cancellous bone alone and showed no signs of pathology on the periapical images. Interestingly, 67% of the teeth did have a buccal cortical plate perforation, but were still not clearly visible on periapical images. They also found that 10% of the teeth examined presented with apical root protrusion beyond the buccal cortical plate. This reinforces that the CBCT is very useful in eliminating the superimposition of anatomical structures, including the radiodense cortical plate, and allowing the clinician to better visualize the pathological changes in periapical bone.
fTyndall and Kohltfarber reviewed the application of CBCT uses and limitations and reinforced the advantages of removing superimposition.The reconstructed scans enable the clinician to visualize periapical pathology, identify and properly locate internal and external resorption, aid in the detection of vertical root fractures, and visualize accessory canals or missed endodontic anatomy. Some of the information interpreted from the scan is taken at face value, whereas some information must be interpreted through an understanding of normal anatomy. For example, when searching for the presence of a mesiolingual canal in a maxillary molar the canal may be visualized on a CBCT scan depending on the voxel size and the existing canal size. Even if the canal is not clearly delineated on the scan, the clinician should examine the root form of the mesial buccal root. When the mesial buccal canal is displaced towards the buccal and the root form is not symmetrical, a mesiolingual canal should be highly suspected. The same is true when evaluating a CBCT scan for a vertical root fracture. Secondary findings such as vertical alveolar bone loss adjacent to a suspected fracture site may be visible on CBCT even when a vertical root fracture is not. In order for a vertical root fracture to be visualized on a scan, the fracture would have to create a large gap between the separated segments. Costa, et al investigated the sensitivity and specificity of the CBCT in the detection of horizontal root fractures. Forty teeth were divided into four equal groups representing either the presence or absence of an induced horizontal root fracture and either the presence or absence of a metallic post. Using a 200 micron voxel size, the teeth were imaged and the scans were interpreted by three oral maxillofacial radiologists.
fThe accuracy was fairly high ranging from 73-88% in the groups without metallic posts, whereas the accuracy fell to 55- 70% when the metallic posts were present. What is worse, the intraobserver agreement fell to 36% when the posts were present. Sensitivity ranged from 0.4-0.75 when a post was present and was as low as 0.55 even without the presence of a metallic post. Although they concluded their results indicated the CBCT is useful in detecting root fractures in endodontically treated teeth without metal posts, the data states otherwise. In fact, multiple observers at multiple times were just as likely to have a false positive type I error as a true positive call. The most likely treatment plan for a tooth with a root fracture is extraction. When an irreversible catastrophic treatment, such as tooth extraction, is relying on an imaging modality to detect or confirm the presence of pathology, it is imperative that it is as accurate as possible. Clinically, CBCT is only an adjunct to the diagnostic process, but the high probability of type I errors could lead to the wrong treatment. In order to understand why this detection error occurs, it is important to understand the acquisition and reconstruction limitations of the CBCT. Jaju et al covered the artifacts in computed tomography acquisition and reconstruction extensively in a 2013 review. All computed tomography scans are inherently more prone to artifacts due to the reconstructed image developed from millions of independent detector measurements. These artifacts can be broken down into four basic categories: physical-based artifacts, patient-based artifacts, motion artifacts, and scanner based artifacts. The first physical-based artifact is termed noise, which is an unwanted randomly distributed disturbance of the signal. As the radiation travels through the patient, the energy can undergo coherent scattering, photoelectric absorption, or Compton scattering. Coherent scattering is change in the direction of the photon due to the photon’s inferior energy level compared to the bound electron in the media, which causes deflection. Photoelectric absorption occurs when the photon energy level is equivalent to or slightly greater than the outer shell electron which causes the electron to be released and the photon to be absorbed. This process repeated many times throughout a medium leads to attenuation of the beam and is the basis for radiation protection equipment. In physics, attenuation is the gradual loss of intensity of any kind of flux through a media. Finally, the Compton Effect describes the process by which a photon causes the release of the outer shell electron, but is not fully absorbed and instead changes direction and continues with a slightly lower energy. All of these forms of scatter can lead to an increase in noise, which is a principle determinant of contrast resolution. Any increase in noise can, and will, lead to a decrease in contrast resolution with a resultant decrease in diagnostic quality. Unfortunately, a leading cause of noise increase is a decrease in the milliamps used. This means that conventional medical CT images tend to show very little noise because they have a high radiation exposure. Whereas the dental CBCT scans tend to benefit from reduced radiation exposure, but in turn have increased noise. The second physical-based artifact is termed beam hardening, which is further divided into a cupping artifact or a streaking artifact.
As individual photons travel through an object, low energy photons are absorbed leaving only very high energy photons to complete the journey. This hardened beam has a higher mean energy, although a fewer number of photons. This causes the sensor to receive greater mean energy than expected in a given area, resulting in a dark streak along the lines of the greatest attenuation. Most current manufacturers of CBCT software try to combat beam hardening through filtration systems, anti-scatter grids, calibration correction, and beam hardening correction software. Most of the filtration systems focus on creating a photon beam profile that has increased intensity in the center. The beam hardening correction software can then be pre-programmed with the correct information to try to reduce areas with a sudden large increase in high energy photons. Anti-scatter grids focus their attention on the receptor instead of the beam. Small lead grids are placed on the sensor that decreases the amount of scatter by only allowing “on-axis” photons to reach the sensor. If a photon deflects off an object, completely changes direction, and heads to the sensor, it is then blocked by the anti-scatter grid based on its trajectory. Unfortunately, this decreases the number of photons reaching the sensor, which in turn inherently increases the noise. If an anti-scatter grid is to be implemented, the milliamps required to decrease the subsequent noise will increase patient exposure. In general there tends to be little advantage to antiscatter grids unless the scans are utilizing a large field of view. Small fields of view, like those utilized in endodontics, tend to have little benefit from the grids.
Finally, manufacturers use software corrections such as calibration correction, scatter correction algorithms, and partial volume artifact algorithms that take into account the filtration and grid usage to combat physical-based artifacts. The next problem encountered is the patient-based artifacts, which tend to lead to physical-based artifacts. As previously mentioned, any hard tissues present in the patient that are outside the field of view in a CBCT scan will create physical based artifacts. Although this includes all hard tissue structures in the head and neck, a major cause is the spine and body of the mandible. The second patient-based artifact is caused by any metallic objects, whether present in the field of view or beyond it. Metallic objects have a density beyond the normal range of the software and tend to highly attenuate the radiation beam. In conventional medical CT imaging, these severe streaking artifacts tend to be only at the level of the metallic object. In contrast, due to the cone-shaped beam in CBCT scans, the severe streaking artifacts occur in all directions from the metal object. This creates a clear limitation to the aforementioned study on fractured teeth. All the teeth in the study were root filled with gutta-percha or contained a metallic post. With a proper understanding of the acquisition and reconstruction process, it is clear that any finding that could be considered a fracture is just as likely to be a severe streaking artifact due to the metallic post or radiodense gutta-percha. Companies like Sirona (Long Island City, NY) have implemented metal artifact reduction software to help reduce the streaking artifact across the reconstructed image. The greater the distance from the metallic object, the better the software is able to correct for the artifact. This creates very limited value in endodontics, as the most important diagnostic information is typically adjacent to the metallic object where there is almost no correction.
The third acquisition and reconstruction artifact is the motion artifact. Conventional periapical imaging occurs in a fraction of a second and leaves very little time for a patient’s motion to be encountered during exposure. Carestream Health, Inc (Rochester, NY) states their CBCT acquisition time is approximately 20 seconds, leaving a large amount of time for a patient to move. With a voxel size of 76 microns in a limited field of view scan, even small patient motion can lead to distortion, ghost images, or double images. It is important that patients are comfortably positioned prior to initiating the CBCT scan to minimize this artifact. The final artifact during acquisition and reconstruction of images involves the scanner itself. Scanner-based artifacts can occur when the detector is out of calibration. Many times these artifacts appear as circular artifacts termed ring artifacts. This should not be confused with the “ringing artifact”, which is a physical based artifact termed the exponential edge gradient effect (EEGE). This effect occurs when there are sharp edges between high contrast structures, which leads to a reduced radiodensity value of the less dense structure. Many times this occurs around restoration margins which can create a false black ring that could be confused with caries. EEGE occurs in the horizontal axis, and is very common in periapical radiography. Due to the three-dimensional nature of CBCT, the EEGE occurs in the vertical dimension as well, which is termed the partial volume effect. Instead, the ring artifact tends to be a darker circle that is associated with the location of the sensor defect.
Current software attempts to correct this ring similar to other artifacts, but it seldom if ever will replicate any pathological process Schulze et al published a review on artifacts in CBCT scans which highlight much of the same information. Here they highlighted the “local tomography” as a major player in CBCT imaging artifacts. Local tomography is the situation in which the limited field of view is actually a very small part of the object being traversed by the photons. As photons travel through the patient they encounter hard tissues, including the spine and other bones, which are not visible in the final reconstruction process. The software must then “guess” as to which photons reaching the sensor are relevant to the reconstruction process and which photons have undergone beam hardening by unknown hard tissues and metal objects. Conventional medical CT images do not have this issue, as the entire patient is in the scan allowing the software to “visualize” and better account for physical-based artifacts. When photons are corrupted by structures outside the region of interest, the true linear attenuation coefficient can not be measured, but instead estimated by the software. This creates beam hardening and scatter from sources that are unknown to the software. Artifacts due to local tomography are especially important to understand in endodontics. It is currently impossible for the software to correct this issue, but these limited field of view scans are ideal for endodontic treatment. Hence, a clinician must understand what parts of the image are likely caused by artifacts and what is likely pathology. Another artifact in CBCT is termed aliasing artifact, which can occur during the sampling or the reconstruction phase. The sampling based aliasing artifact can be due to the divergent nature of the cone beam. As the beam travels it spreads out in every direction. Hence, objects closer to the beam will receive more photons per area than an object a greater distance away. The detector itself can then receive an under-sampling of a certain area, leading to aliasing artifact. By nature, this will lead to less artifact and higher imaging quality in the central plane.
The image quality will then degrade as a function of the distance from the central plane. Since this cause of aliasing artifact is a function of distance, there is a minimal effect in limited field of view scans with proper patient orientation. The reconstruction cause of aliasing artifact is due to the computational limitation on the reconstruction process. In a perfect reconstruction, the intensity of a voxel would reflect the exact volume of the received photos for a sampled area. Although a
76μm voxel size is very small, it may not be small enough to properly represent everything that exists within the voxel’s volume. Due to the computational limitation on the number of samples per second and the limited voxel size, a crude interpolation must be inserted. This aliasing artifact can lead to the appearance of Moiré patterns which diverge from the center towards the periphery and are likely due to under-sampling. Even though software algorithms attempt to correct many of these artifacts, they can never be perfect. This is because the reconstruction process is always following the acquisition phase where the error occurs. Unless technology can be developed to alter the acquisition methodology, the reconstruction will always have artifact. Despite the numerous limitations artifacts create, CBCT scans have still been shown to be quite useful. Scarfe et al presented a literature review to investigate the CBCT uses specific to endodontics. Due to the new nature of CBCT technology, there was an absence of high level randomized clinical trials. Through the remaining low level studies they were able to highlight several applications for select cases. Published research highlighted the usefulness of the CBCT for preoperative assessment of tooth morphology, periapical pathosis, root resorption, and the post operative assessment of fill quality and healing. Although no imaging technique can be expected to be 100% accurate, the majority of the published data focused on the complementary effect the CBCT had to current imaging techniques, and that it should not be a replacement for standard digital radiographic applications.
The main advantage they found for the CBCT were specific situations in which understanding the anatomical special relationships could alter or facilitate the diagnosis, treatment plan, or treatment. Researchers have previously attempted to use imaging techniques to aid in the diagnosis of periapical lesions.Conventional periapical imaging techniques have proven to be unreliable in differentiating between a periapical cyst and a periapical granuloma. Guo et al attempted to evaluate CBCT scans for key radiological characteristics that are assumed to be cystic qualities. These included being located at the apex of the tooth, well-defined cortical border, radiolucent shape is curved or circular, internal structure is radiolucent, displacement and resorption of the roots of adjacent teeth with a curved outline, and perforation of the cortical plate. After the three evaluators examined the CBCT scans, the patients underwent periapical surgeries for histological examination, which was considered the gold standard. They found that the most accurate correlation resulted when scans showing four or more of the key radiological characteristics were deemed a cyst, whereas teeth with three or fewer should be classified as a granuloma. Using this criterion the overall accuracy was .77, and their conclusion was the CBCT image can provide a moderately accurate diagnosis between cyst and granulomas. Unfortunately, there are major design flaws in the study that create a large limitation to their findings. The first problem lies in the very small sample size of only 10 lesions confirmed as a cyst histologically. All three examiners had a sensitivity of diagnosing a cyst of 0.6, which means the false-negative rate was 0.4, well within the probability of chance. On the contrary, the specificity ranged from 0.77-0.92 for the 26 cases of granulomas. A receiver operative characteristic (ROC) curve was created for each of the possible number of key radiological characteristics. For example, a ROC curve was created for radiographic findings called a cyst when presenting with only one key characteristic. Then waiting to call the lesion a cyst until it presented with at least two key characteristics, and so on. The data presented on the ROC curves was drastically variable, mainly due to the limited sample size of ten. According to the logic behind their study, requiring more key characteristics should inherently decrease the sensitivity of cyst diagnosis by increasing the number of false negatives. If a CBCT scan presents with all six characteristics it should more likely be a cyst than a scan presenting with one, yet would miss many cysts presenting with five or less characteristics. This reveals the underlying problem with the initiation of their key characteristic model.
The six characteristics for cystic diagnostic criteria were based on the radiological presentation of periapical cysts in periapical radiography, which has proven to be unreliable and inaccurate. Due to the high specificity when four or more criteria are required, a different conclusion should have been rendered. When a CBCT scan presents with fewer than four of the key characteristics, it can provide a moderately accurate confirmation for the lack of a cyst. Even this conclusion, although more accurately reflecting the data presented, is hindered by the small sample size. Another focus of CBCT imaging technology is the possibility of evaluating postoperative healing. As discussed previously, when pre-operative CBCT scans were evaluated for bone density and determining volume and then compared to a post-operative recall CBCT scan two years after treatment, they showed a significant difference in bone density Here they concluded that a CBCT can be used as a reliable indicator of endodontic radiographic healing, although there was no comparison to the current periapical imaging standards. This raises the question as to what is the best technology to evaluate outcomes. Peters et al addressed this question by investigating different imaging techniques to detect periapical changes. Currently, and throughout most of the modern endodontic history, conventional periapical radiographs are the standard used to evaluate periapical changes. With digital technology, two radiographs can undergo subtraction radiography to gain a better understanding of subtle changes in radio-density. When reviewing CBCT uses, Peters et al commended the images for removing prevalent problems incurred with periapical imaging including superimposition and distortion. Here they recommended using CBCT scans for healing assessment of periapical osseous lesions, conditions of the maxillary sinus, status following endodontic surgery, hard tissue deposition during regenerative procedures, and evaluation of horizontal root fractures. If the CBCT is to become the standard imaging technique for detecting periapical changes, it is possible that a new outcome classification system is needed. As discussed previously, Garcia de Paula-Silva et al suggested that the CBCT is more sensitive to detecting apical periodontitis than conventional periapical radiography. When clinical outcome studies begin to use the CBCT instead of periapical images for apical periodontitis resolution, it is likely that more teeth will be moved from a completely healed category to a healing category. Peters et al in a literature review found that the negative predictive values for periapical images compared to histological findings ranged from 25-75%. This indicates that it may even be the majority of endodontically treated teeth deemed radiographically to be negative of apical periodontitis in which apical periodontitis is still present histologically. The CBCT will detect more cases of subtle apical periodontitis than periapical imaging, but this usefulness may be a detrimental limitation to a patient if the clinician does not have a full understanding of outcomes. According to Peters et al, current literature shows the success rate determined by full resolution of a finding is 10-40% lower in CBCT imaging than conventional periapical radiography. The CBCT evaluation
should be an adjunct in clinical decision making. If it becomes a major determinant for the threshold into retreatment or surgery, patients may be subjected to potentially unnecessary procedures. The debate as to the consequences of not treating subtle chronic apical periodontitis is not resolved in the literature. Currently there are no clinical studies that truly support or negate if apical periodontitis persisting after root canal treatment can either predispose or lead to systemic complications such as coronal heart disease. As CBCT imaging technology reveals a higher prevalence of post-treatment apical periodontitis, further studies will need to investigate the possible sequelae and should establish guidelines to aid the clinician on treatment intervention thresholds. Similar to all radiographic techniques, a possible limitation to the use of CBCT imaging is radiation exposure. It is important for the clinician to quantify and qualify the different types of radiation exposure in order to weigh the risks and benefits for each patient. Radiation dosimetry is categorized in three dose units; the radiation absorbed dose, the equivalent dose, and the effective dose. As radiation is created and sent towards the patient it has a specific amount of energy measured in joules. The target patient tissue has a specific mass which is measured in kilograms. The radiation absorbed dose is the absorbed energy per unit mass which is joules per kilograms, also known as the Gray (Gy). Although the Gray is useful in quantifying the amount of absorbed energy, it can not be used to compare dosages between patients because it fails to account for what type of radiation is present and the target tissue’s degree of sensitivity to irradiation. This damaging nature is mathematically represented by the radiation-quality weighting factor. When this factor is multiplied by the radiation absorbed dose it represents the equivalent dose, measured in Sieverts (Sv). The equivalent dose allows for the comparison of the radiobiological effects. These effects can be further multiplied out by different tissue weighting factors which converts the equivalent dose into the effective dose, or the whole body dose.
Once the effective dose is established, different imaging techniques and different parts of the body can be more realistically compared. The effective dose created by CBCT imaging varies depending on the targeted patient area, the field of view, and the different manufacturers. Larger fields of view correspond with more sieverts delivered to the patient, anatomical structures with greater radio-density require more exposure, and each manufacturer varies in their claimed μSv effective doses. In endodontics, when only a small field of view is required, the effective dose can be as low as 7.3 μSv, comparable to a conventional periapical image which requires approximately 5 μSv of effective dose exposure. Although the exposure can range significantly, CBCT imaging comes no where close to conventional medical grade CT scans. Although the scan times tend to range from 10-40 seconds, the radiation beam is pulsed resulting in 2-5 seconds of actual exposure time. Medical grade CT scans undergo continuous exposure during the acquisition phase resulting in significantly more effective dose exposure to the patient. Pulsated exposures create a large number of mini-exposure projection images which are reconstructed by the software to create a volume consisting of over one million voxels (29). As the micron slice thickness decreases, the number of voxels increases exponentially, and the amount of radiation exposure required to maintain clear resolution increases as well. The resolution is itself another limitation of CBCT imaging when compared to conventional periapical radiographs. Independent line pairs range from 15-20 plus line pairs per mm-1 in periapical imaging, whereas the CBCT images only have a spatial resolution of 2 line pairs per mm-1. As the technology continues to advance, hopefully the software will be able to increase the spatial resolution in CBCT imaging.
With the ability to eliminate the superimposition of anatomical structures, the clinician gains a better understanding of the true anatomy. Root form can be evaluated for the presence or absence of complex tooth anatomy including extra canals, dilacerations, and resorption. Prior to any surgical procedure, a CBCT image will give the clinician the ability to evaluate the location of nerve canals, the maxillary sinus, or alveolar bone width in relation to the tooth in question. When a CBCT scan is made prior to endodontic treatment, diagnosis is more accurate when compared to surgical histological findings, and treatment plans are changed to reflect the proper diagnosis. With all of these advances the CBCT brings to endodontic imaging, diagnosis and treatment planning, it is imperative that the clinician understands the limitations. All images must be interpreted with a full comprehension of the radiographic artifacts that will be present in every CBCT image in order to reduce the risk of over diagnosis. Future studies will also need to evaluate the existence of persistent radiolucent periapical findings on CBCT scans and guide the clinician to proper treatment planning and intervention thresholds.
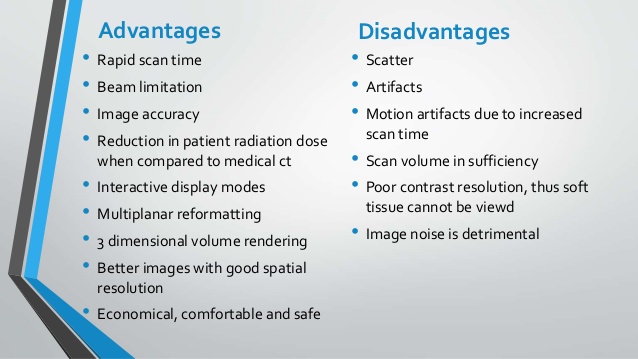
Cone Beam Computed Tomography (CBCT) Uses and Limitations