Breast Cancer
According to the American Cancer Society, breast cancer is the most commonly detected type of malignant tumor in women. For 2014, more than 230,000 new cases are expected to be diagnosed, amounting to 29% of the total number of new cancer cases in women. From these cases, about 40,000 will result in the patient’s death. As a consequence, it is considered a major health problem.
It is encouraging to note, since 1990, breast cancer mortality rates have declined as a consequence of the possibility of detecting the disease at an early stage and because of the improvement in treatment options. Early detection of breast cancer has been made possible by improved screening programs that aim at finding breast lesions before the diseases have spread to other parts of the body
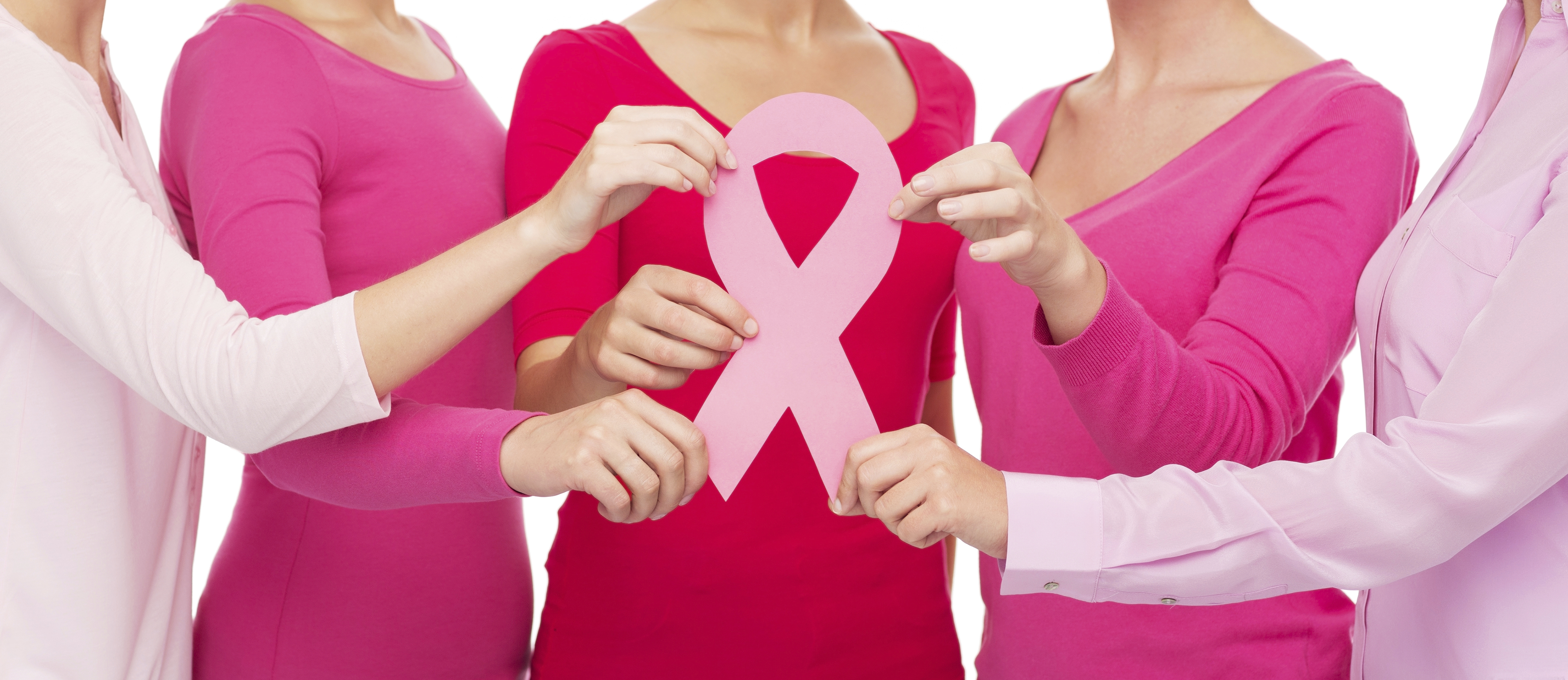
Breast Cancer
X-ray mammography
X-ray mammography is the primary imaging modality for screening asymptomatic women. The American Cancer Society recommends an annual mammography study for women at or older than age 40. This imaging modality is based on the detection of x-rays transmitted through the breast. The detection method can be radiographic film or, more commonly, a digital detection device. Many studies have demonstrated that the early detection of breast cancer by means of screening programs based on mammography augment the treatment options and the possibility of their success. Evidence shows that mammography screening studies reduce breast cancer mortality. X-ray mammography suffers from an important limitation: its projective nature. The cumulative absorption and scattering of the x-rays along their way to the detector by the volumetric structure of the breast is registered in a two dimensional image.Thus, the complicated internal structure of the breast reduces the conspicuity of any abnormality that might exist within it. As a result, an estimated 10% to 20% of malignant lesions in the breast go undetected using mammography. The sensitivity of mammography is further reduced, to about 69% in one study, in women with radiographically dense breasts, i.e., those that contain a high fraction of glandular tissue and ducts.This problem is particularly important in women under age 40 who tend to have radiographically denser breasts than older women, and who have a propensity to develop more aggressive types of cancers than other women, leading to a poorer prognosis than for women over 40.

mammography
Pulse-echo ultrasound for breast cancer detection and diagnosis
In the context of breast cancer detection and diagnosis, the role of pulse-echo ultrasound scanning has been mainly as a supplemental examination for the differential diagnosis of suspicious findings. An example of a helpful finding is the anechoic appearance of cysts compared to the echogenic nature of solid masses. Ultrasound is also used as a guiding tool during biopsy procedures that utilize minimum invasion. Recently, various studies have demonstrated that by performing conventional ultrasound sequentially after negative screening mammography (in those cases where screening mammography was negative) the rate of the detection of malignant lesions can be increased by 22%. This increased detection power occurs mainly in women with breasts in which the fraction of glandular composition is above 50% (dense and highly dense breasts according to the categorization from the American College of Radiology). However, this comes at the expense of increasing the rate of false positive detections, i.e., cases deemed suspicious under imaging studies, but the pathological results indicate a benign lesion. In reviewing multiple studies about the advantage of performing bilateral ultrasound in cases where screening mammography revealed a suspicious finding, Nuthacker et al. found that from cases in which ultrasound suggested a suspicious finding, only about 15% were cancers. Thus the number of biopsies in which the lesion was deemed benign was about 85%. This means that 85 out a hundred patients were subjected to the psychological stress that cancer suspicion and the biopsy procedure itself cause when they only had benign (or no) disease. Thus the overall consensus is that the emotional distress and the economic burden of additional benign biopsies that result from complementing x-ray mammography with conventional ultrasound are not justified by the gain in detection power. In addition, it has not yet been demonstrated that sequential ultrasound screening leads to a reduction in cancer mortality. One of the main factors leading to the limited specificity of conventional B-mode ultrasound is that the appearance of a lesion in an ultrasound image depends on the skill of the operator, the scanner configuration, and the transmission path that the acoustic pulse traverses on its way to the lesion. These factors lead to inter- and intra-observer variability in the evaluation of these images, that reduces the objectivity of the imaging modality. Aiming at standardizing the interpretation of sonographic features in ultrasound images as well as improving patient management, the ACR introduced in 2003 the Breast Imaging Reporting and Data System (BIRADS) for evaluation of breast lesions.9-11 This system defines a common lexicon to describe various features related to the appearance of masses and their surrounding tissue in the ultrasound image. These features are presented in Table 1 Malignancy is usually associated with masses that have spiculated margins, an irregular shape, and a “nonparallel” orientation. The Positive Predictive Value of the margin, shape, and orientation features (the ratio of the number of detected cancers based on a particular feature to those classified as cancers) were 86%, 62%, and 69%, respectively. Lesions with circumscribed margins, oval shape and aligned parallel to the surrounding tissue layers tend to be benign. The Negative Predictive Values (ratio of the number of benign cases classified as benign using a particular feature to the total number of benign classifications) of these features were 98%, 84%, and 78%, respectively. The BIRADS lexicon descriptors provide a final classification scheme which assigns the lesion to one of seven different groups, described in Table 1.

Table1 Breast Imaging Reporting and Data System – Final assessment categories
In spite of the existence of this standardization scheme, studies evaluating the inter-observer variability of the use of this classification have reported limited agreement on both the use of the sonographic features and the final outcome classification. In 2009, Abdullah et al presented a retrospective evaluation of the assessments of 267 breast masses made by five radiologists who had been using the BIRADS terminology for at least 2 years. The authors used the Landis and Koch interpretation of the Aickin statistic to quantify inter-observer agreement in the following categories: poor agreement
), fair agreement (
), moderate agreement (
), substantial agreement (
), and excellent agreement (
). Results showed that the observers achieved only fair agreement in describing the margins of the lesion, which is the parameter with the highest positive predictive value for malignancy. Other descriptors showed moderate to substantial agreement. Less agreement was observed 6 in lesions with diameters smaller than 0.7mm, which limits the early detection and diagnostic power. More importantly, a greater disagreement was observed in the final outcome categorization (
, fair disagreement), particularly among categories 4a, 4b, and 4c. The importance of this finding lies in the fact that these categories span a probability of malignancy from 3 to 94%, thus including a large variety of lesion features. Biopsy is recommended in all of them. In the case of the intra-observer variability, Calas et al reported moderate to substantial agreement among evaluations of BIRADS features separated by 3 and 6 months by each of 8 radiologists with 5 to 23 years of experience in evaluating ultrasound images of the breast. Other studies have found similar trends. Thus, increasing the diagnostic power of pulse-echo ultrasound is expected to reduce the number of biopsies with negative results. This could ultimately reduce the emotional distress of patients and the amount of resources and time devoted to the detection and diagnosis of breast cancer

Breast Imaging Reporting and Data System
Quantitative Ultrasound

Quantitative Ultrasound
The development of a particular disease often affects the regional cellular microenvironment. As a consequence, tissue microstructure (glands, collagen bundles in connective tissue) suffers modifications in its acoustic properties. Based on this premise, various research groups have explored methods to offer a quantitative assessment of these acoustic properties aiming at tracking how their values under healthy circumstances are affected by different pathological processes. These methods are commonly referred to as Quantitative Ultrasound (QUS). The goal of QUS is to estimate acoustic properties of tissue and use the values as system- and observer-independent diagnostic parameters. This would reduce the subjectivity of the sonographic analysis and, therefore, increase its specificity. QUS has greatly evolved in the sixty years since the seminal work by Wild and Reid, which aimed at differentiating breast cancer from benign lesions based on simple characteristics of the radio frequency (RF) echo signal such as the number of echoes and the average area beneath the demodulated RF(envelope, or “A-mode”) echo signal trace. As of today, the various QUS techniques and methods can be grouped into three general modalities: backscatter-based QUS, flow-based QUS, and elasticity-based QUS. The first modality aims at extracting information about the structural and physical properties of tissue microstructure from the statistical analysis of the scattering process. Some backscatter techniques are performed in the time domain, such as deriving envelope statistics or carrying out image texture analysis. Other backscatter techniques are performed in the frequency domain using spectral analysis of the RF signals. The second QUS modality encompasses Doppler-shift based techniques (color Doppler,power Doppler), widely used to study blood flow and the presence of new vascularity in tumors. Finally, the goal of elasticity-based techniques is to quantify the elastic and viscous properties of tissue.The estimation of parameters in each of the QUS groups starts by the selection of a Region of Interest(ROI) over which the parameter will be analyzed. Within the ROI, a Parameter Estimation Region defines the minimum amount of data used for parameter estimation. An ROI may contain one or many parameter estimation regions depending on the task to be performed. This was succinctly defined by Lizzi et al. as: bulk estimation or parametric imaging. Bulk estimation is a “large estimation region” task because the ROI includes usually one parameter estimation region. It aims at comprehensively gathering values of tissue properties to be used in tissue characterization or classification. This mode is often used for the analysis of ex vivo tissue samples, such as in D’Astous and Foster In parametric imaging, the parameter estimation region is moved across the ROI in a raster scan fashion to estimate the parameter of interest in various spatial locations. In this way, a 2D array of local values of the parameter of interest is obtained and then color-encoded to create a final image. Figure 1 illustrates the areas defined by the ROI and the parameter estimation region when creating a parametric image. The B-mode image on the right of Fig. 1 was created from backscattered echo data acquired in vivo from a human breast carcinoma.The ROI outlined in the image might include a lesion or a section of it and the surrounding tissue. Within the ROI, a parameter estimation region (PER) is defined and includes a set of adjacent segments of echo signal data, or the volume of tissue they represent, that is used to compute a QUS parameter. For the estimation of spectral-based QUS parameters, such as the attenuation coefficient or the backscatter coefficient in Fig. 1, the PER can include one or more power spectral density (PSD) estimation windows indicated by each of the dashed-framed boxes in the diagram on the left. This is because the estimation of the attenuation coefficient requires the quantification of the power loss over the propagation length (
-estimation length) defined by the axial span of the centers of the PSD estimation windows.

Figure 1 Description of a parameter estimation region (PER) from which one estimate of the attenuation coefficient is obtained. Each power spectral density (PSD) estimate is assigned to the center of the spectral estimation window, indicated by a dot. The PER width is defined by the number of adjacent scan lines it includes, and its length is defined by the α-estimation length plus one spectral estimation window length. The abbreviation “est.” stands for estimation