Introduction To Wireless Medical Implants
Wirelessly networked systems of implantable medical devices endowed with sensors and actuators will be the basis of many innovative, sometimes revolutionary therapies. Artificial pancreases, i.e., implanted continuous glucose monitors wirelessly interconnected with adaptive insulin pumps, could ease the life of many type-1 diabetic patients. Post-surgery sensors will detect changes in the microenvironment involved with the surgical procedure (pressure, Ph, white cells concentration, LDH enzyme) to prevent infections or ischemias caused by tissue perfusion. At an even smaller scale, wirelessly controlled nanorobots could detect and eliminate malicious agents and cells inside biological tissues, e.g., viruses and cancer cells, enabling less invasive and less aggressive treatments. Other fascinating applications include functional electrical stimulation, a particular type of neurostimulation that tries to restore motion in people with disabilities by injecting electrical currents to activate nerves innervating extremities affected by paralysis; or distributed pacemakers for advanced cardiac resynchronization therapy. Existing as well as futuristic applications of wireless technology to medical implantable (as well as wearable) devices will grow into a new market segment that several analysts are already starting to refer to as “The Internet of Medical Things”.
As of today, existing wireless medical implants are connected through radio frequency (RF) electromagnetic waves. RF-based solutions tend to almost-blindly scale down traditional wireless technologies (e.g., Wi-Fi, Bluetooth) to the intra-body environment, with little or no attention to the peculiar characteristics and safety requirements of the human body. We contend that this may not be the right approach. The human body is in fact composed up to 65% of water, a medium through which radio frequency (RF) electromagnetic waves do not easily propagate. Since tissues absorb the RF waves, much higher transmission power is needed to establish reliable communication links, thus reducing the battery lifetime, or, equivalently, increasing the battery size of an implantable device. Energy efficiency, miniaturization, and battery duration are major concerns in the domain of implantable medical devices, where a surgical procedure is, in most cases, needed to replace the batteries of implants. Additionally, the general public perceives microwaves as potentially dangerous – the World Health Organization classifies RF waves as “possibly carcinogenic to humans”. Last, RF-based technologies raise serious concerns about potential conflicts with existing RF communication systems that can unintentionally undermine the reliability and security of the intra-body network, and therefore the safety of the patient. Finally, RF communications can be easily jammed, i.e., intentionally disrupted by artificially generated interference, or eavesdropped by malicious agents, raising significant privacy and security red flags, and a risk for the patient.
Given these limitations in this work, we propose and investigate the use of ultrasonic waves as an alternative carrier of information in human tissues. Ultrasonic waves are acoustic waves with frequency higher than the upper threshold for human hearing (nominally 20 kHz). Compared to radio-frequency (RF) electromagnetic waves used in Bluetooth or WiFi, ultrasonic waves have the following key benefits for use in implantable biomedical devices:
• Significantly lower absorption by human tissues (e.g., 8−16 dB for a 10−20 cm link at 1MHz, vs 60−90 dB at 2.45GHz as used in Bluetooth). As a consequence, tissue heating is much reduced, which makes propagation safer for humans. Moreover, lower transmission powers are needed, and therefore implantable battery-powered devices may last longer or be smaller in size.
• The FDA also allows much higher intensity for ultrasonic waves (720mW/cm2) in tissues as compared to RF (10 mW/cm2), i.e., almost two orders of magnitude higher. Therefore, wireless recharging of batteries through ultrasonic waves has the potential, with appropriate design, to be orders of magnitude faster than with RF.
• From a communication theoretic perspective, multi-path is easier to resolve because of the lower propagation speed of sound; one can build small transducers that operate at low frequencies, and these are easier to couple to human tissues than RF antennas (which instead need to operate at high frequencies).
• From a security perspective, ultrasonic communications in tissues cannot be easily eavesdropped or jammed without physical contact.
• Finally, there are no electromagnetic compatibility concerns with a crowded RF spectrum – in the words of the FDA, “An increasingly crowded RF environment could impact the performance of RF wireless medical devices”
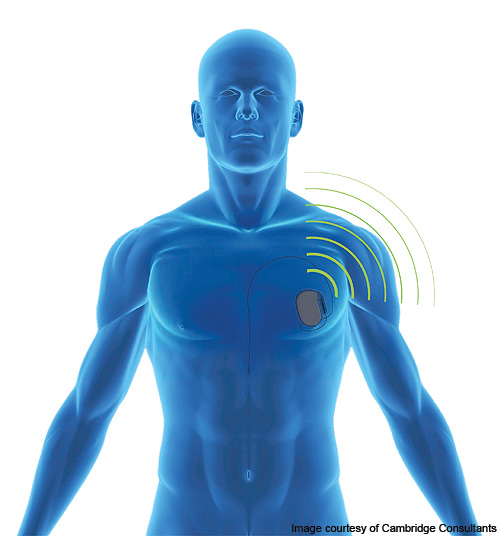
Introduction To Wireless Medical Implants